All the Carcinogens We Cannot See
We routinely test for chemicals that cause mutations. What about the dark matter of carcinogens—substances that don’t create cancer cells but rouse them from their slumber?
December 11, 2023
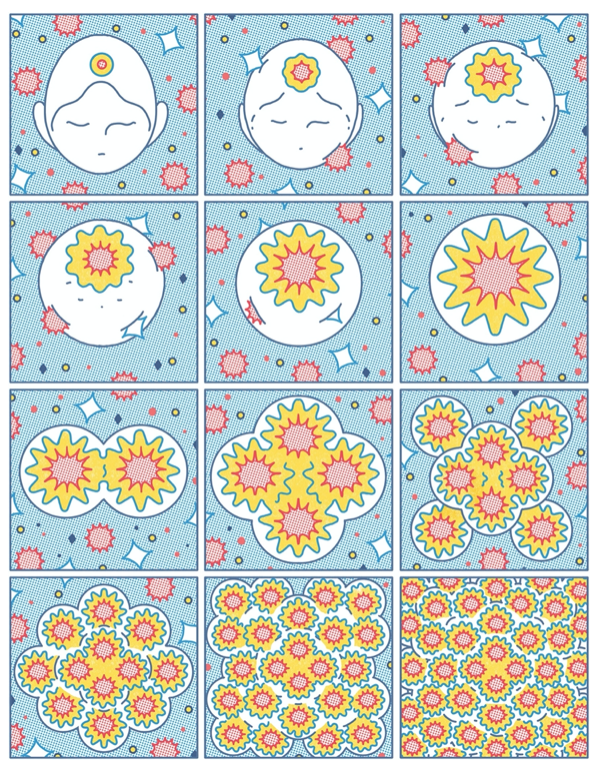
In the nineteen-seventies, Bruce Ames, a biochemist at Berkeley, devised a way to test whether a chemical might cause cancer. Various tenets of cancer biology were already well established. Cancer resulted from genetic mutations—changes in a cell’s DNA sequence that typically cause the cell to divide uncontrollably. These mutations could be inherited, induced by viruses, or generated by random copying errors in dividing cells. They could also be produced by physical or chemical agents: radiation, ultraviolet light, benzene. One day, Ames had found himself reading the list of ingredients on a package of potato chips, and wondering how safe the chemicals used as preservatives really were.
But how to catch a carcinogen? You could expose a rodent to a suspect chemical and see if it developed cancer; toxicologists had done so for generations. But that approach was too slow and costly to deploy on a wide enough scale. Ames—a limber fellow who was partial to wide-lapel tweed jackets and unorthodox neckties—had an idea. If an agent caused DNA mutations in human cells, he reasoned, it was likely to cause mutations in bacterial cells. And Ames had a way of measuring the mutation rate in bacteria, using fast-growing, easy-to-culture strains of salmonella, which he had been studying for a couple of decades. With a few colleagues, he established the assay and published a paper outlining the method with a bold title: “Carcinogens Are Mutagens.” The so-called Ames test for mutagens remains the standard lab technique for screening substances that may cause cancer.
Scientists, including Ames, realized from the start that the test wasn’t a comprehensive method for catching carcinogens. Epidemiologists were learning, for instance, that exposure to certain estrogen-like chemicals, such as diethylstilbestrol (DES), increases the risk of vaginal, cervical, and breast cancer. (Toxicologists found similar results in mice and rats.) Yet DES wasn’t obviously mutagenic in cell cultures; its cancer-causing mechanism is still being explored, but probably involves driving the growth of hormone-responsive cells or changing the expression of cancer-linked genes. In time, additional classes of carcinogens were added to the list. One feature of cancer cells is that they avoid detection by the immune system. And so compounds that suppress the immune system, such as cyclosporine, were recognized as cancer-promoting chemicals, even though they don’t cause mutations in DNA.
But there were mysteries in carcinogenesis that continued to puzzle toxicologists. Earlier this fall, I discussed the issue with Allan Balmain, a cancer geneticist at the University of California, San Francisco. Balmain, who is in his seventies, was born in Wick, Scotland, and still speaks with a Scottish burr. He wore a cardigan that had a noticeable hole but matched the cornflower blue of his darting eyes. “Virtually every standard model for finding and classifying carcinogens has relied on what it does to the cancer cell,” he told me. Balmain had come to believe that we were thinking far too narrowly. He was hinting at unsolved mysteries in cancer epidemiology. The incidence of colorectal cancer in young men and women in the United States, for example, has nearly doubled since 1995. In certain pockets of the world, lung-cancer rates in young, non-smoking adults are rising dramatically. Although researchers have advanced various theories about why, there’s a sense that some cancer-inducing factors elude our apparatus of detection. It’s as if there were dark matter lurking in the cosmos of carcinogens.
We were standing on a catwalk that led to Balmain’s lab, within an airy, glass-lined atrium overlooking Third Street, in Mission Bay. A few blocks away, a colossal Mark di Suvero sculpture, a multi-ton steel assemblage with four ladder-like legs and outstretched beams, rose above a grassy hill. I was struck by how much the landscape made the art. Without the grass and the hill, the piece might be taken for one of the construction cranes that hover over the campus. Balmain, too, was talking about context. Cancer cells live and grow surrounded by normal cells—buried in a landscape of normal tissue. Shouldn’t we extend our focus to the larger ecosystem where cancers arise?
He guided me to his office, a space so clean and organized that it looked like housekeeping had just visited. In a 2020 study, he and a team of researchers exposed mice to twenty chemicals that are known or suspected human carcinogens. Tumors formed, and the team analyzed their DNA. “These are potent, well-established cancer-causing agents,” Balmain told me. “You would expect the tumors that grew to be splattered with mutations.” That’s because DNA-disrupting chemicals have signature mutagenic effects, found not just in the specific genes linked to cancer but throughout the genome. But in the case of seventeen of the chemicals, there was no clear link between them and the mutations. “It was a head-scratching result,” Balmain said. “And so I’ve really started wondering if they are changing the cells that surround the cancer.”
In the eighties, not long after Balmain started researching cancer, the field was dominated by a step-by-step “multi-hit” model of carcinogenesis. A normal cell acquires one genetic mutation, and then another, and then another, moving, gene by gene, toward becoming a malignant cell. Perhaps one mutation overactivates a gene that promotes cell division, another mutation inhibits a gene that triggers cell death when abnormalities are detected, and a third sabotages a gene specializing in DNA repair. “That’s absolutely true for some cancers, and that’s how the story ran,” Balmain told me. But at least one mouse study, conducted in the forties, demonstrated that the genesis of cancer could also follow a different trajectory. “It was an experiment that didn’t fit the standard model of carcinogenesis,” Balmain said.
In the experiment, two researchers working at Oxford, Isaac Berenblum and Philippe Shubik, assembled a group of mice, clipped a patch of hair on each rodent’s back, and painted the patches with DMBA, a cancer-linked chemical that was found in coal tar. Yet only one animal in thirty-eight developed a malignant lesion. When the researchers added some slicks of croton oil to the same area, the results were startlingly different. (Croton oil, a blistering, inflammatory liquid extracted from the seeds of an Asian tree, was used as an emetic and as a skin-sloughing exfoliant.) Now malignant tumors bloomed, appearing on more than half the mice. The sequence mattered. Reverse the schedule of application—croton oil first, tar after—and there were no tumors.
It was as though the known carcinogen, DMBA, had primed the cell and the croton oil had catapulted it toward malignancy. Berenblum and Shubik saw croton oil as a “promoting” agent: here was a new kind of carcinogen that, it appeared, acted through an inflammatory response. The idea that inflammation could lead to cancer wasn’t new. In the eighteen-seventies, the Viennese surgeon Alexander von Winiwarter had proposed that cancer was a consequence of an incompletely healed wound. But in what sense was croton oil a carcinogen? The mice painted only with croton oil hadn’t developed tumors. It passes a standard Ames test on bacteria, and a more sensitive version of the Ames test that uses fruit-fly cells. It’s negative even with animal cells. There’s no evidence, in short, that it causes DNA mutations.
So what was its contribution, and why did it do its malign work only after the coal-tar chemical was applied? Yes, it caused inflammation, but plenty of things do. A staph infection in the skin produces a potent inflammatory state, and yet it doesn’t cause skin cancer. The mystery perplexed cancer biologists for decades. What if this mechanism wasn’t an incidental curiosity but a major source of disease which we were only starting to understand? And, if so, what kind of substance irritates its victims to death?
Scientific investigations, like detective stories, take place within an epistemological system, a way of knowing. To identify the murderer, we might need to first identify the method of murder. But sometimes this presents a more complicated puzzle than we might anticipate; the weapon may involve a confluence of factors. In Arthur Conan Doyle’s “The Adventure of the Speckled Band,” Sherlock Holmes is on guard for a mysterious killer who can seemingly slip through doors. He sits awake in a room, waiting nervily by a ventilation shaft. The weapon turns out to be a venomous snake; it climbs through the shaft via a rope and poisons its victim. Yet the snake isn’t the singular cause: it’s necessary but not sufficient. The murderer has to whip the snake into a frenzy—inflame it—before it will attack. In “The Hound of the Baskervilles,” the titular creature doesn’t maul its victim but frightens him to death. The hound is lethal because it has been painted with a phosphorescent substance, and because of a specific context: the victim is terrified by a legend that says his ancestors were haunted by a supernatural monster. Conan Doyle was drawn to these additive scenarios because they expanded the complexity of a mystery.
For decades, chemical irritants presented carcinogen hunters with a structurally similar problem. These agents may work only in combination with other chemicals; like a hound on the moor, they, too, might depend on the victim’s history of prior exposure (DMBA first, croton oil next). And, as with Conan Doyle’s creaturely killers, they may also depend on multiple causes: the irritant promotes the development of tumors, but only after an initiator has been applied. How, then, might we devise a test for a substance when it acts only in concert and in context?
Not long after Berenblum and Shubik published that paper, a doctor named Irving Selikoff opened a medical clinic in Paterson, New Jersey, a largely working-class city. His was a blandly modern office: veneer panels, a curved Formica-topped desk, a few chairs. In the postwar years, factories were starting to close, but Paterson’s Union Asbestos and Rubber Company factory, which produced asbestos insulation material, was still operating. And members of an asbestos-workers union enrolled in his practice.
Selikoff was particularly attuned to lung diseases. By the early fifties, he had learned to treat tuberculosis using the antibiotic isoniazid (for which he and a couple of colleagues would win a Lasker Award). Soon, he began to notice lung problems—most prominent, deposits of calcium in scarred, inflamed lesions—in patients with asbestos exposure. “These men came home each day covered with the whitish fibers of asbestos—on their clothes, in their hair, in their lunch pails,” the Times later reported. “Sometimes they brought home samples of the fireproof product they made for their children to play with.”
As the years passed, the cases took a more ominous turn. Selikoff, confirming research done in Britain and Germany, noted that the workers were succumbing to a rare, lethal form of cancer that typically spread through the lining of the lung: mesothelioma. X-rays lit up the white shadow of the cancer coursing through the back and the bottom of the lung. The tumors often invaded the spine and the chest wall, and led to agonizing deaths. By the early sixties, Selikoff had collected data on a cohort of six hundred and thirty-two men who had worked in the insulation factory, some for many years. Among these men, Selikoff documented forty-five cases of lung cancer and mesothelioma—seven times more than the expected number. The incidence of stomach, colon, and rectal cancer was three times higher than expected.
Even as asbestos was identified as a major occupational carcinogen, however, scientists struggled to understand how it might cause cancer. The research remains inconclusive. In a study published in 1977, researchers exposed various strains of bacteria to asbestos fibres, and didn’t find that the fibres were associated with mutations. The toxicologists persisted. In one study, researchers added several other chemicals to asbestos and finally found bacterial mutants. In another, asbestos-induced chromosomal abnormalities were detected in animal cells. Yet another study found that mice injected with asbestos developed cancer, but with a latency period that seemed inexplicably long, given the material’s potency as a human carcinogen. And a study of a cohort of Turkish villagers exposed to one type of asbestos came to the opposite conclusion: sensitive tests revealed no increase in DNA damage. It was obvious that asbestos exposure raised your risk of cancer; it wasn’t obvious how. Like croton oil, asbestos seems to act as a promoter. Generate a mutation first, and then add an irritant, and a cell is propelled toward becoming a tumor.
In science, a clamorous consensus often begins as a whisper. Long before cancer was understood as a disease of mutated genes—indeed, long before the terms “gene” and “DNA” entered our scientific vocabulary—a London physician named Percivall Pott wrote about the cancerous scrotal sores that were turning up in chimney sweeps, who, since early childhood, had been “thrust up narrow, and sometimes hot chimneys, where they are bruised, burned, and almost suffocated.” Although the condition was initially attributed to a venereal disease—what better explanation for a poor man’s genital lesions than his presumed promiscuity?—Pott conjectured, in a 1775 essay, that the “soot-wart” was caused by chronic exposure to soot particles, which lodged in the scrotal ridges.
It’s tempting, of course, to extrapolate Pott’s findings on soot-induced cancers to cancers caused by cigarette tar in humans. But it has been challenging to test cigarette tar or smoke in a standard Ames test. Cigarette smoke certainly contains mutagens—more than sixty have been identified—and, by extension, carcinogens. Yet a 2023 study that examined the characteristic fingerprints of DNA damage caused by cigarette smoke in human lung cancers revealed an unexpected result. Among cancer specimens from smokers, ninety-two per cent had the telltale fingerprint of cigarette-smoke-induced genetic damage—they had the mutations associated with the DNA-damaging substances in smoke. But about eight per cent lacked this kind of damage, suggesting some other mechanism by which those cancers may have arisen.
The fact that, in almost one in ten cases, we can’t identify a clear mechanism for the development of lung cancer even in smokers gives us all the more reason to think that we may be missing a plenitude of cancer-causing agents. The multi-hit model tells us about what’s happening within a cell, but Balmain knew that a cell is not an isolated spaceship floating between planets. That’s why he came to suspect that some of the “hits” have to do not with the cancer cell but with the tissue milieu in which the cancer cell finds itself. The snake, venomous as it is, must still be whipped to provoke its attack; the hound must be painted and set to roam the moor.
“Wait, wait,” Balmain said as I got up to leave his office. “I need to show you a picture.” He pulled up an image on his computer. A few years ago, a postdoctoral researcher in his lab had obtained a genetically altered line of mice; when they were given a chemical trigger, a powerful cancer-causing gene would be activated in their skin cells. But when the trigger was administered very little happened. “And that’s exactly what happens with most of these genetic mouse models,” he said. “You trigger genes implicated in human cancers, and then you wait for months before you get tumors. Guess what happened when we activated our cancer gene? Just bugger all. The mutant cells were there, all right, but no tumors.”
Next, the researchers in his lab made a linear incision in the skin of the mice. Still no tumors. And then—in that liminal space between serendipity and intentional experimentation—Balmain’s team stumbled on a peculiar result. The researchers had put three surgical staples at the incision site. (The experiment had been monitored carefully by vets to minimize animal suffering.) Poorly healing wounds—chronic inflammation—had formed around the three sites. And precisely three tumors had grown there.
Balmain used his pen to point out the tumors on the computer screen: “One. Two. Three.” He went on, “It’s just as with Berenblum’s experiments. We had primed the cells to become malignant, but they remained dormant.” They formed tumors only after the chronic irritation had catapulted them out of normalcy. “The mutant cells just lie there,” he said. “It’s the inflammation that awakens them.”
I looked through Balmain’s office window. It was 6:30 p.m., and the cars on the road were starting to lock next to each other like Tetris blocks. Uber’s headquarters, a hulking glass building a few streets away, would soon be emptying out. Before long, the air outside would smell like traffic.
“But what if that irritation is caused by an environmental insult, like air pollution?” I asked. The surgical staples weren’t mutagens, of course, but they precipitated tumor formation. By the same logic, the irritant chemical could be a substance that we eat, or a chemical we expose our children to, or something in the environment that we unsuspectingly breathe. It probably wouldn’t be caught by an Ames test or by any standard toxicology study. Could there be a universe of promoters that we’d been missing because we hadn’t been looking in the right place?
“Ah, that you’ll have to ask Charlie Swanton, in London,” Balmain said.
Charles Swanton’s lab is at the Francis Crick Institute, near King’s Cross. Like Balmain’s research center in San Francisco, the building has an enormous open atrium, with cascading walls of glass, a bank of elevators, and connecting catwalks. Cancer labs come in clones, I thought.
And clones are what Swanton works on. In cancer biology, a “clone” is a group of genetically identical cells. Imagine an enormous family of cells: a great-grandfather, his children, their children, their children. Some may have acquired mutations of their own, through the vagaries of cell division; they’re called subclones. But the population is “clonally related”—that is, there’s a genetic lineage that connects them all.
Wiry and rail thin, Swanton looks like an athlete, his body a coil of sinewy intensity that seems to have dispensed with all excess, including the hair on his head. His seminal work, carried out over the past decade, concerns tracking clones in human cancers as tumors grow. Cancer, as he conceives it, is a disease of clonal competition. Which clones become dominant as cancer evolves? His research has provided a granular description of cancer’s evolution. A one-centimetre tumor contains something like a hundred million cells. “These hundred million cells are all descendants from one cell—that is, they are clonally related,” Swanton explained. “But by the time that tumor develops it already contains myriad individual clones.”
This is a chilling duality of cancer: each individual cancer comes from a single cell, and yet each cancer contains thousands of clones evolving in time and space. Treating or curing cancer involves tackling this incredible degree of genetic diversity. It’s a clone war. And the clinical relevance is obvious. Clones that develop mutations conferring resistance to anti-cancer therapies are the ones that flourish and form metastases. “You can’t always stay ahead of all those clones,” Swanton observed—underlining the importance of trying to prevent tumors from forming in the first place.
In June, 2019, Swanton flew to South Korea to give a talk on clonal evolution in cancer. Jet-lagged, exhausted, and more than a little sleepy, he finished his talk and walked to a reception in the hotel lobby. “You know the routine,” he said. “Chitchat, a glass of wine, nibbles, and then to bed.”
A young Taiwanese doctor flagged him down. He’d listened closely to Swanton talking about mutations in lung cancer. The doctor showed him a map of fine-particulate air pollution across the planet, and then a map charting the incidence of lung cancer in nonsmokers. “I think I might have woken up a bit,” Swanton said. There were areas of striking correlation. “In southern China, in Taiwan, in the areas north and south of Hong Kong, you could see the juxtaposition of the two phenomena.”
That’s where the mystery began. So far, air pollution has not been shown to be notably mutagenic. Tiny airborne particulate matter—designated PM2.5, for its size (no greater than 2.5 micrometres, about thirty times smaller than the width of a human hair)—enters the small airways of the lungs. But the stuff doesn’t detectably damage DNA; it may be that whatever potential mutagens are present don’t reach sufficient concentrations for that. Swanton wondered what might underlie the correlation between air pollution and lung cancer among nonsmokers.
Back in London, Swanton handed the project to three researchers: William Hill, a Welsh running enthusiast; Emilia Lim, a high-spirited Canadian whose Singaporean grandmother never smoked but succumbed to lung cancer; and Clare Weeden, an Australian devotee of detective stories. When lung cancer occurs in people who have never smoked, the malignant cells often carry a mutation in a gene known as EGFR. Lim examined the link between air pollution and EGFR-mutated lung cancer, working with a team of epidemiologists and using data sets from the U.K., South Korea, and Taiwan. In each of the three countries, she found, the higher the level of air pollution the higher the incidence of EGFR-mutated lung cancer. The U.K. Biobank, which tracks the health of some five hundred thousand volunteers, generated additional data, helping to confirm a link between air pollution and nonsmoking-related lung cancer.
I met Hill and Weeden at the Crick Institute on an overcast morning. (Lim was setting up a new lab in Canada.) It was about eleven, and the lab was whirring with activity. Hill walked me to his lab bench. It was piled with a precarious tower of boxes—each containing dozens of glass slides with slices of tissue—that invited thoughts of the bookcase that toppled on poor Leonard Bast in “Howards End.”
Hill reached into a drawer and pulled out a vial filled with a coal-black sludge. “That’s a solution of suspended particles of dust and soot,” he explained. “It’s liquid air pollution.”
I shook the vial, watching the particles rise and settle. It was as if someone had made a hideous snow globe with the grime wiped from my windows in New York.
“Careful,” Hill said. “It comes from the National Institute of Standards, and the liquid contains a defined composition of particles collected from an air-polluted urban environment. It’s about fifteen hundred pounds per bottle. Pricey soot.”
Hill, Lim, and Weeden had begun their experiments with mice that were genetically engineered to have a mutant EGFR gene that could be turned on by a chemical trigger. After the researchers activated the cancer-linked gene in the mice’s lung cells, the animals developed sporadic tumors—a low background hum of malignancy. “And then came the tricky part,” Hill said. The researchers instilled various doses of the dusky air-pollution liquid in some of the mice’s lungs. Ten weeks later, when the mice were examined, the data were striking: as the dose of the mixture had increased, so, too, had the frequency of lung tumors. At the highest dose (and, thus, the highest number of particles instilled), there was a nearly tenfold rise in the number of lung tumors.
What was the mechanism? Hill, Lim, and Weeden used gene sequencing to compare the tumors that had arisen in the non-treated mice and the tumors in the PM2.5-treated mice. In both kinds of tumor, they identified the EGFR mutation that had been purposely activated and, as expected, a few additional ones that had arisen spontaneously.
But it’s what they didn’t find that was crucial. “The mutation numbers in the control mice were not significantly different from those in the treated mice,” Hill said. Whatever had caused the tenfold increase in the number of tumors in these mice wasn’t producing new mutations inside the cancer cells. Hill and his colleagues suspected that the effects of the liquefied air pollution were found outside the cancer cell. “And so we went back to the tissue, dissecting out the tumors, cutting slices, and looking under the microscope,” Hill said. The lungs of the mice that had been exposed to the air pollution, they found, were full of inflammatory cells.
Hill walked me to a microscope on his desk. The facility had dozens of powerful scopes, but this one was the sort of basic instrument that you might find in a school lab. I looked through the lens at a wafer-thin section of a mouse lung. “This was from one of the mice that we treated with a high dose of air pollution,” Hill told me. In the center of the slide was a disk-shaped lung tumor packed with misshapen malignant cells, but the tumor was like a raft in a seething ocean of inflammation. The researchers’ analysis provided a detailed description of the inflammatory cells. They found a particular kind of macrophage—a cell so named because it’s large (macro) and eats (phago) foreign particles—jump-starting an immune response by pumping out a potent inflammatory signal, interleukin-1 beta. When the interleukin-1 beta was blocked with an antibody, the effect of air-pollution exposure was attenuated. And when Hill, Lim, and Weeden reran their experiment with immune-deficient mice, the effect of air pollution disappeared. The macrophages, and their signals, were somehow promoting tumor development.
“But now the puzzle really deepened,” Weeden said. We had moved from the lab upstairs to a circle of couches in the lobby on the ground floor, where Weeden could watch her four-month-old daughter, who was asleep in a stroller with Clucky the duck, her favorite stuffed toy. We spoke quietly to avoid disturbing the baby. “If she moves, Clucky will quack, and she’ll wake up,” Weeden said. “Once she’s awake, it’s all over.”
What perplexed Hill, Lim, and Weeden involved the human epidemiology of lung cancer: if PM2.5 was not a mutagen but was merely awakening the growth of a preëxisting mutant cell, where did that original mutant cell come from? Weeden continued to talk quietly, gesturing at the sleeping child. It felt vaguely conspiratorial, as if we were offending an old orthodoxy. “It’s a little crazy, isn’t it?” she said. “For nearly four years, we had been the outsiders in a genetics lab, working on pollution sludge while everyone else was sequencing genes and looking for clones. And now it was reversed. We were the clone hunters. We were back in the thick of genetics, hunting for mutant clones.” There was a sharp clang from the elevator bank. Thankfully, the baby continued to sleep.
If the original mutant cells existed before PM2.5 exposure, then the genetic tools developed over the decades to track rare clones should be able to find those cells. So the team examined pieces of normal lung tissue from human patients, using state-of-the-art “deep sequencing” methods, making thousands of passes through thousands of DNA strands to achieve a high level of precision. EGFR mutant cells turned up in a significant minority of cases. The researchers calculated that the incidence of these cells was roughly one in half a million, which sounds minuscule until you remember that lung cells may number in the trillions. It’s the nature of these mutant cells to go cancerous—if they receive the right nurture.
Hill, Lim, and Weeden aren’t the only researchers to have found potentially cancerous cells lying dormant in tissues. In 2015, a team of scientists studied cells from the eyelid, an area of skin routinely exposed to UV light. (The tissue came from patients who’d had “eyelid lift” surgery.) Roughly a fifth to a third of the cells carried skin-cancer-driving mutations, and clones carrying some of these mutations had expanded, suggesting positive selection. Yet none of these patients had overt skin cancer. Such research suggests that healthy people may have a cadre of potentially cancerous clones.
“And that’s the answer,” Swanton told me when we spoke later, a jolt of intensity in his voice. “The simplest explanation is that people who don’t smoke, and those who do, have preëxisting mutant cells, albeit at a very rare frequency, in their lungs. In the case of air-pollution-induced cancer, the PM2.5 particles activate immune cells to create an inflammatory milieu.” And it’s in this boggy cesspool of soot-spurred inflammation that the mutant cells find their footing.
Swanton’s model sits athwart the standard model for carcinogenesis. In this case, the tumor doesn’t evolve mutation by mutation (although, as the tumor grows, additional mutations can still accumulate). The original mutant clones are already there, sleeper cells awaiting activation.
“Then why aren’t we bursting with cancer?” I asked, recalling Balmain’s slide. I’d had minor surgery a few months before, and the surgeon had left nine staples in my skin. Why weren’t nine tumors growing out?
“There’s some bad luck involved—you have to have a bad cell at the bad place at the bad time, and over a long period,” Swanton said. “There may be hereditary influences, too, or gene-environment interactions that dampen or accelerate the growth of some clones.” With carcinogenesis, as with so much in life, the right combination of nature and nurture is required.
The paper in which Swanton’s lab presented its findings about air pollution and lung cancer appeared in Nature earlier this year, and it ends on an ominous note: “Our data suggest a mechanistic and causative link between air pollutants and lung cancer, as previously proposed, and substantiate earlier findings on tumour promotion, providing a public health mandate to restrict particulate emissions in urban areas.” The risk extends to a vast population. “Ninety-nine per cent of the world’s population is exposed to levels of air pollution that exceed the safety guidelines published by the World Health Organization,” Hill told me. Smoking increases your risk of lung cancer far more than air pollution, but the number of people exposed to air pollution is so vast that the over-all toll of each may be similar. Swanton has estimated that air-pollution-induced lung cancer kills seven or eight million people every year.
The issue of Nature in which the air-pollution paper ran featured, on its cover, a photograph of a smog-choked New Delhi, my home town. As I write this, in November, the city is in partial lockdown because the level of air pollution has reached a limit considered dangerous for human habitation. I recall a tragicomic moment from my youth when the government decided to erect a pollution meter atop one of the busiest traffic intersections in New Delhi. Cars, scooters, and trucks belched smoke as they snaked through the crossroads. Factories spewed dark, ash-laden air into the sky. Trees, blackened with soot, stood like silhouetted ghosts along highways. One morning, looking up at the air-pollution meter, I realized that I couldn’t read the numbers. The glassy front was black. The device for measuring soot had been obscured by soot.
“Let me tell you a fact that only a handful of epidemiologists remember,” Swanton told me. When the British epidemiologists Richard Doll and Austin Bradford Hill were trying to identify causes of lung cancer, they had narrowed their suspicions to two main candidates. One was cigarette smoking, and they’re still celebrated for having established the link between cigarettes and cancer. But, Swanton pointed out, their classic paper mentioned another cancer correlate—proximity to major roadways, gasworks, industrial plants, and coal fires, and thus, by extension, exposure to high levels of air pollution. “I wonder how the history of cancer prevention may have been written differently if biologists had the tools then to investigate that cause,” Swanton mused.
In the end, as Holmes aficionados know, the detective never did trap the murderer in “The Hound of the Baskervilles.” Stapleton, the villain, wanders through a woolly, impenetrable fog, is sucked into a bog in the moor, and asphyxiates to death. He leaves the barest signs of his existence: a boot and a tub of the phosphorescent paint that he used on the hound.
I asked Balmain how we might devise a trap for carcinogens that work by changing the tissue milieu—the cancerous cell’s context. Could there be an Ames test for environmental inflammogens? We use the word “inflammation” colloquially, as if we understand what we are saying. But dig a little deeper and the word becomes a clown car of meanings. Is it the chronic irritation of an autoimmune disease? The lingering residue of a postviral syndrome? A nonhealing wound? Given the exquisite specificity of the kind of inflammation that Swanton’s team had identified—mediated, it appeared, by macrophage-produced interleukin-1 beta—what test would capture just that form of it?
“I’m really not sure,” Balmain confessed. But there are hints about where to start looking. He has been working with a team that is attempting to create such a test. One version relies on using a special type of “organoid”—in this case, three-dimensional clusters of skin cells and immune cells cultured together. (My own laboratory works on similar cancer organoids.) In principle, the chemical irritant might induce immune cells to produce an inflammatory cascade, and that inflammation, in turn, might make the cancer cells grow.
Or carcinogen hunters might study macrophages. They might, for instance, expose macrophages to chemicals that would jump-start the particular immune response, mediated, in part, by interleukin-1 beta. Or they might look in animal or human bodies for an immune-signal signature, as a surrogate for the sort of inflammation that promotes cancer. Signals indicating an imbalance of immune cells might predict a “pro-cancerous” state, perhaps leading to more intensive monitoring for prevention. That approach wouldn’t necessarily identify the culprit, at least at first, but it would provide evidence of the misbehavior—like finding the tub of phosphorescent paint on the trail of the hound.
The walk from Swanton’s lab to St. Bartholomew’s Hospital takes about half an hour. It was a pleasant afternoon in London, so I decided to foot it. I wandered onto Greville Street, heading toward the hospital. About a mile and a half to the west was the London School of Hygiene & Tropical Medicine, where Austin Bradford Hill, working with Doll, at the Medical Research Council, had followed a cohort of more than forty thousand doctors for twenty-nine months and published the classic study about smoking and lung cancer. A little less than two miles east were the wards of the London Hospital, where Muriel Newhouse and Hilda Thompson had reported on patients with mesothelioma who had been exposed to asbestos.
I sat down on a roadside bench to read the Nature paper again. “The one way to identify a great work of literature,” a friend and avid reader once told me, “is that the person who begins the novel and the person who finishes it are never going to be the same. The novel changes you.” The same might be true for great work in science. It fundamentally changes the way you see the world. Swanton’s team had wrestled the mystery down using epidemiology, toxicology, immunology, and human genetics, and then pulled them together into a causal, biologically plausible mechanism for carcinogenesis. It represents one of the most elegant reconciliations between disciplines that I have encountered in science.
The asbestos-linked tumors that Selikoff had found? Well, asbestos, most likely, is a promoter more than a mutagen, and its carcinogenic properties may be a consequence of the irritation that it produces. The fibres might summon macrophages and other immune cells that produce scarring and inflammation in the lungs, and this irritation might awaken preëxisting malignant clones. Cigarettes? The chemicals in tar cause mutations. Fine soot particles are the irritant. And the nicotine makes it addictive. A cigarette, in short, achieves a troubling trifecta: inflammogen, mutagen, and addictogen, all rolled conveniently into a thin cylinder.
A young man pushed past me in a haze of menthol, pulling on a vape pen. Scalding, vaporized particles of a chemical mixture wafted into his body. I wondered what a biopsy of his lung might reveal. A passing motorcycle coughed out a trail of black exhaust, and I wished I were wearing a mask. I found that I could think about the world around me only in terms of chemical irritants and inflammations. By now, it was about three in the afternoon, and I realized that I had reached the front gates of St. Bartholomew’s, where Pott had reported on those pernicious soot warts. It was also where Swanton had done some of his medical training. And, I recalled, where Watson had first met Holmes.
Environmental carcinogens have been notoriously difficult to find because toxicology, an experimental science, has to keep pace with epidemiology, an observational one. It’s a frantic chase: human studies are often throwing up puzzles that bacterial and animal tests cannot explain. And it’s often hard to recapitulate “environments” in laboratory settings. (Who knew that the National Institute of Standards and Technology sells bottled air pollution?) Fortunately, the fields are converging; we’re getting smarter at catching new culprits. But we’re just beginning to grasp how many might be out there.
By the time I left St. Bartholomew’s, I felt an urgent need to revisit the world of Conan Doyle. I hailed a cab and, in the spirit of completing the journey, rode to Baker Street. ♦
Published in the print edition of the December 18, 2023, issue, with the headline “Sleeper Cells.”